
Strongly coupled system between atoms and a Fabry-Pérot (FP) cavity. The cavity is composed by two super-mirrors with curvature radii of 100 mm and the length is hundreds of micrometers. The reflectivity of the two mirrors are 99.990% and 99.999%, respectively. Thus, photons in the cavity mode can be reflected back and forth between the two mirrors for about tens of thousand times before decays into the free space. Due to the tiny mode volume, the electric field associated with the single photon is very strong and can saturate the atomic transitions. When single atom is placed in the cavity mode, the photon would be coherent transferred between the atom and cavity mode at frequency around 10 MHz. The system is called strongly coupled cavity QED system when the photon transfer frequency is bigger than the photon decay rates. The system is then very sensitive to small change of the photon or atom numbers. Theoretically, the system is capable to sense the number change of photon or atom smaller than one. This kind of interaction will induce a series of novel quantum effects. With the aid of cold atoms, cavity QED has drawn extensively attentions in the researches of fundamental physics, and it will play important role in the study of quantum optics and quantum information processing. There are several cavity QED setups in our group. Cold cesium atoms with μK are manipulated in the cavity mode by laser cooling and control technology. The interaction between atom and cavity can be precisely controlled and the quantum states and dynamics can be monitored by recording single photons emitting from the cavity mode. The collective radiation of multiple atoms, quantum optics with atoms and photons, and new devices for quantum information processing are currently under investigation.
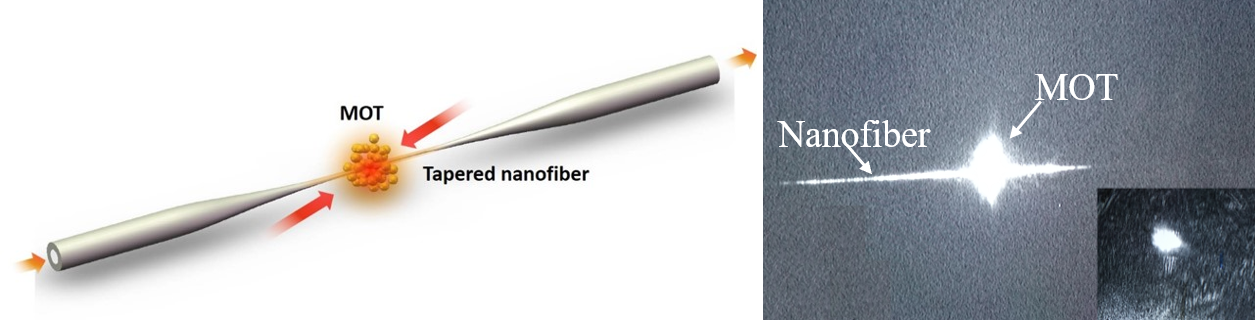
Nanofibers have been a versatile photonic platform which can confine and shape the light. Nanofibers can enhance light-matter interactions, control single emitters and provide high photon collection efficiency. Nanofibers have been widely applied in applications ranging from photonic devices to optical sensing to quantum optics. We have built an experimental system for controlling and detecting cold atoms based on nanofibers. A home-made heating-stretching system is used to fabricate nanofibers in our group. Nanofibers are fabricated by heating and stretching a regular single-mode fiber, where the regular single-mode fiber is narrowed down to a subwavelength diameter. Evanescent fields are localized at the interface between the nanofiber with a high refractive index and air with a low refractive index. The evanescent fields are enhanced dramatically. Therefore, the enhanced evanescent fields are confined strongly in the vicinity of the nanofiber surface, which can enhance the interface between evanescent fields and matter such as atoms, color centers in diamond, quantum dots, molecules, metal particles, and graphene particles. As a hybrid photonic platform combining nanofibers and cold atoms, this system has been used for quantum optics and single emitter detection. The system will play a significant role in precision measurement, quantum information processing.

As one of the most important tasks in quantum research field, quantum computing has been explored for decades with various of physical systems. Optically trapped neutral single-atoms system, which has the advantages of the long coherence time and good scalability, has been regarded as one of the most promising systems to achieve the quantum computation. The foundation of the natural atom-based quantum computing is the resolvable single atom array. In the very recent time, the demonstration of full-filled 2D or 3D resolvable trap arrays and high-fidelity quantum gates via Rydberg interaction accelerated the researches on the neutral atom-based quantum computation. By using the home-made long-working-distance and large-numerical-aperture objectives we have obtained the blue- and red-detuned optical dipole traps and successfully loaded single atoms. The trapped single atom is then used to encode single qubit and the high-fidelity initialization, gate operation, and readout of the qubit has been demonstrated in our group. The single-qubit system can be further scaled up to a large-size multiple qubits system for quantum computation. The combination of the scaled multi-qubit system and FP cavity has the potential to develop a computing node in quantum networks.
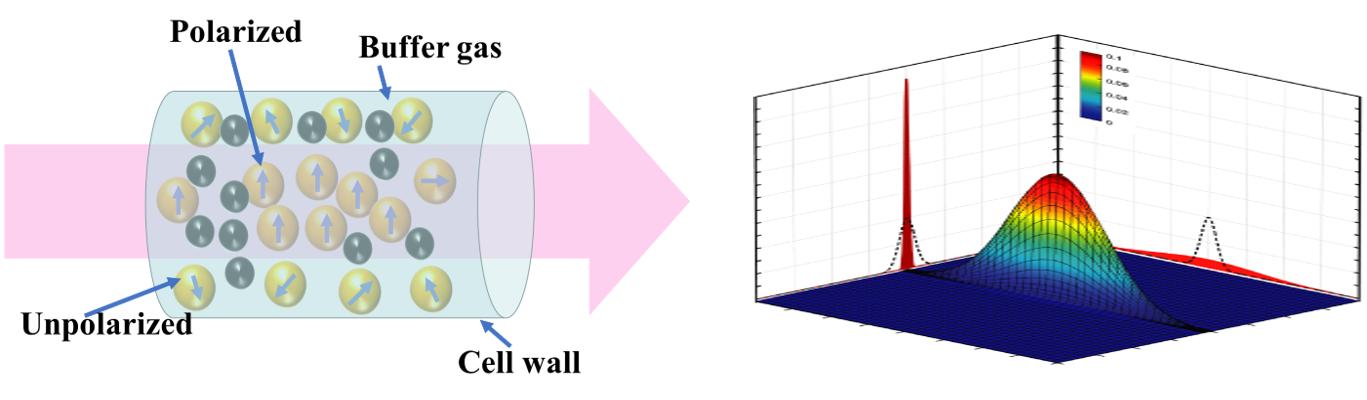
Quantum Metrology uses quantum resources to implement precision measurement and can break the bound of standard quantum limit. Various of quantum resources has been experimentally produced, however, how to use them became one of the problems. As one of the typical quantum resources, the squeezed state has been adopted to detect the gravitational wave in LIGO and tiny space vibrations can be precisely extracted. Similarly, the precise measurement of magnetic field plays important role in many fields, for example, mineral exploration, geological detection, biomedicine and aerospace. Along with the better understanding of the interaction properties between light and atoms, magnetometer based on the atom-field interaction has been proved to have much better sensitivity and developed in the past half century, At present, the sensitivity of atomic magnetometer is the highest in the whole world, which can reach even the scale of 0.1fT/sqrt(Hz). The atomic magnetometer can be used to measure near-zero magnetic field, non-zero magnetic fields, geomagnetic fields, scalar and vector magnetic fields, and it has a wide application prospect. The classical limit for the sensitivity of the magnetometer is the standard quantum limit of the photons and atomic spins. By using squeezed state of atomic spins or light field the sensitivity can be further enhanced. We have obtained magnetometer based on hot atomic vapor and squeezed light field. The combination of both would allow us to explore the influence of squeezed state on the sensitivity of magnetometer.